No products in the cart.
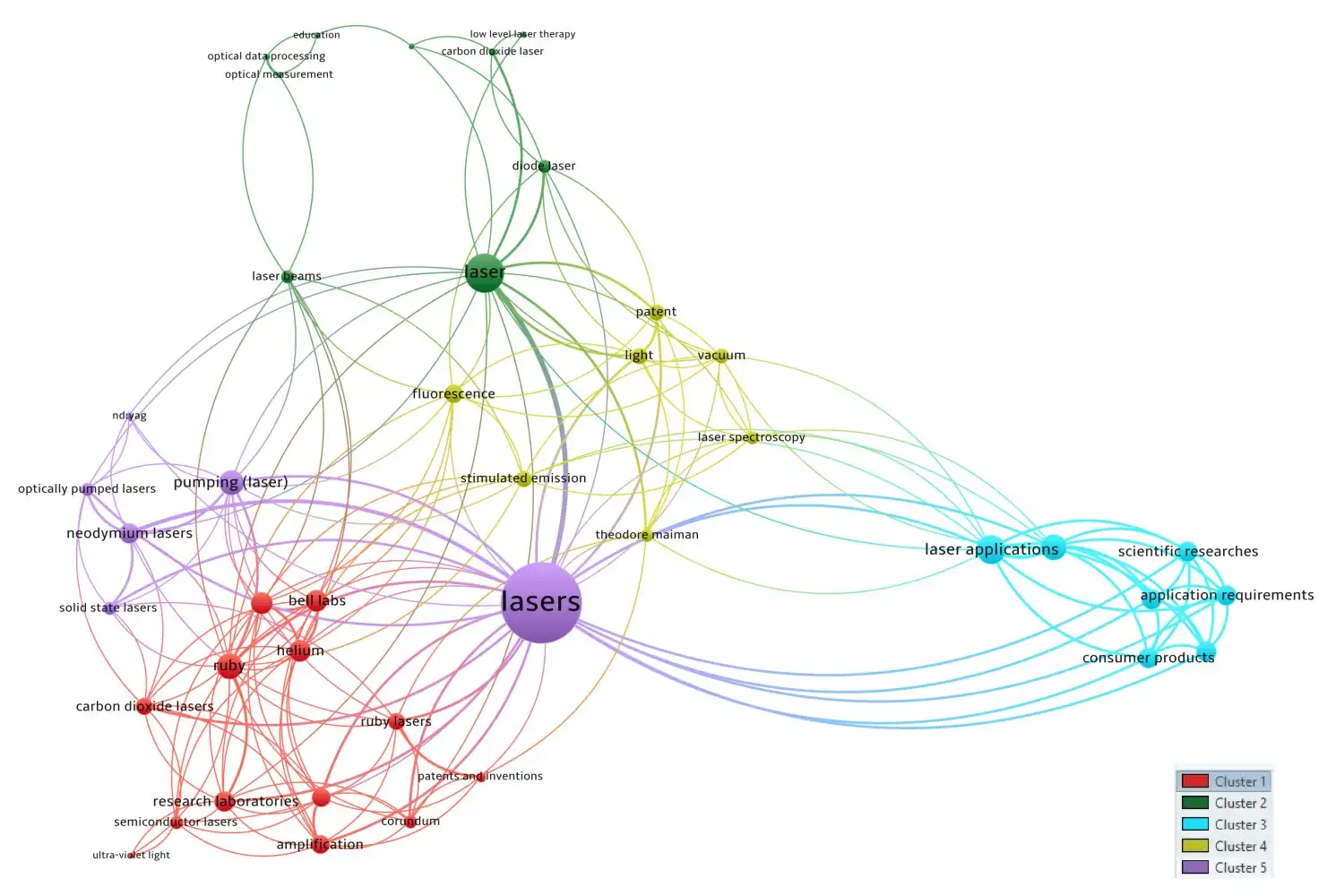
Theodore Maiman, Light & Laser: Quo Vadis?
Contents
I. Abstract
The field of laser, light and energy encompasses various areas of research and applications, including photonics, renewable energy sources, lighting technologies, and optical materials. In recent years, the integration of artificial intelligence (AI), data science, and statistics has brought significant advancements to this field, revolutionizing how we harness and utilize light and energy. This literature review provides a comprehensive overview of the use of AI in the field of light and energy, highlighting their applications, methodologies, and challenges. This article presents a comprehensive bibliometric analysis of trending topics in light and energy data from a Theodore Maiman perspective. The objective is to identify and analyze clusters of related topics using co-occurrence network analysis. The study aims to provide valuable insights into the applications of artificial intelligence, data science, and statistics in the field of light and energy.
II. INTRODUCTION
III. LITTERATURE REVIEW
A. AI and Machine Learning in Light and Energy
B. Data Science Approaches in Light and Energy
C. Statistical Analysis in Light and Energy
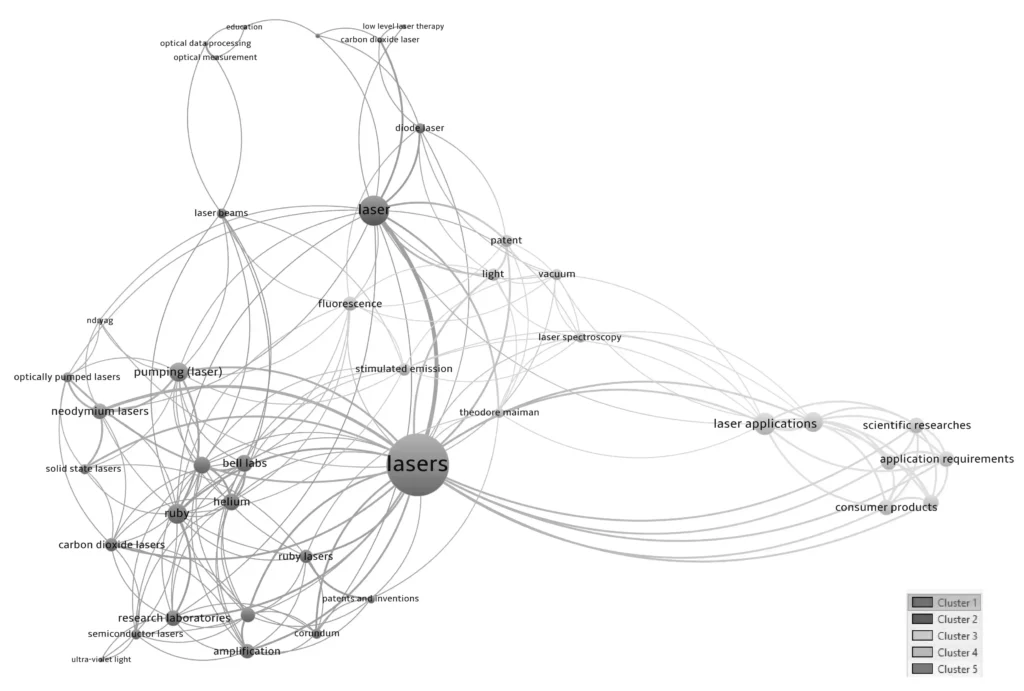

IV. METHODOLOGY
V. RESULTS
- Cluster 1
- Cluster 2
- Cluster 3
- Cluster 4
- Cluster 5
VI. LIMITATIONS
VII. CONCLUSIONS
VIII. REFERENCES
- Drexhage, K. H. (1974). Influence of a dielectric interface on fluorescence decay time. Journal of Luminescence, 8(4), 693-701.
- Duarte, F. J., & Hillman, L. W. (Eds.). (2012). Dye laser principles. Academic Press.
- Maiman, T. H. (1960). Stimulated optical radiation in ruby. Nature, 187(4736), 493-494.
- Miller, D. A. B. (2009). Optical interconnects to electronic chips. Proceedings of the IEEE, 97(7), 1166-1185.
- Nugent-Glandorf, L., & Lombardi, J. R. (2019). State-of-the-art advances in ultrafast lasers. Chemical Reviews, 119(16), 10754-10828. doi:10.1021/acs.chemrev.9b00157.
- Pollock, C. R., & Yariv, A. (1977). Coherence and quantum properties of light sources. Reports on Progress in Physics, 40(6), 827.
- Schawlow, A. L., & Townes, C. H. (1958). Infrared and optical masers. Physical Review, 112(6), 1940-1949.
- Sliney, D. H., & Dennis, J. E. (Eds.). (1994). Safety with lasers and other optical sources: A comprehensive handbook. Plenum Press.
- van Gemert, M. J. C., Welch, A. J., Pickering, J. W., & Sterling, A. M. (Eds.). (1995). Laser applications in medicine and biology. Springer Science & Business Media.
- Yariv, A., & Yeh, P. (2006). Photonics: Optical electronics in modern communications. Oxford University Press.
- Yen, W. M., & Shionoya, S. (Eds.). (2003). Phosphor handbook. CRC Press.Yen, W. M., & Shionoya, S. (Eds.). (2003). Phosphor handbook. CRC Press.
Author
-
Milena-Jael Silva-Morales is the Founder & Director of Ecolonical LAB, an independent research lab specializing in data, AI, and territorial systems. A systems engineer with a Ph.D. in Urban & Territorial Systems and over 13 years of experience in R&D&I, she focuses on FAIR data governance, AI ethics, and interdisciplinary methodologies for water, energy, and biodiversity systems.
View all posts
This article is governed by the Ecolonical Open Knowledge License (EOKL Lite V1). This license explicitly prohibits the use of its contents for AI model training, dataset integration, algorithmic processing, or automated decision-making systems. Unauthorized computational aggregation, reproduction beyond permitted terms, and any use conflicting with open knowledge principles are strictly restricted.
For legally binding terms, compliance obligations, and permitted exceptions, refer to the License Usage Policy.
Under specific conditions, this content aligns with the Creative Commons Attribution-NonCommercial-ShareAlike 4.0 International License. However, any AI-related processing, direct commercial exploitation, or automated derivative work remains subject to EOKL Lite V1 restrictions.
Leave a Reply